HOW A CAPACITOR WORKS
It's handy to know the effective reactance (resistance) of a capacitor
at the operating frequency of the circuit. If we assume 100MHz, the
resistance is as follows:
Capacitance |
reactance @100MHz |
reactance @2kHz |
5.6p |
300R |
|
10p |
166R |
|
22p |
75R |
|
47p |
35R |
|
1n |
1R6 |
|
22n |
much less than 1R |
3k7 |
1u |
----- |
82R |
How a capacitor works depends on where it is placed. A 22n across the power rails will be like a small
battery equal to the voltage of the supply, but with an internal
resistance of less than one ohm.
When a battery has a low internal impedance, a high current can be taken
without the voltage dropping. You may not think the oscillator circuit
takes a high current but if the average is 10mA, there will be times
when the circuit requires 20mA, and times when it needs 1mA.
If the voltage dips when the circuit is trying to charge a capacitor,
for example, the capacitor will not get charged to its maximum.
This is what happens with the circuits above. As soon as you put a 22n
across the battery, the output increase a small amount.
Not only does the output increase, but the increase stays throughout the
life of the battery, especially when it is getting flat. So the 22n
across the battery is very important.
A ceramic capacitor is able to supply this tiny amount of charge very
quickly and this is needed as the circuit is working at 100,000,000
times per second.
An electrolytic is not able to supply a tiny amount of charge at this
fast rate and so an electrolytic is not suitable for the supply
"decoupler."
A decoupler is the name given to any capacitor that is placed across the
supply rails to suppress spikes or prevent the effects of one stage from
interfering with another stage. It "decouples" or "separates."
When a capacitor is used to "couple" one stage to the next, such as the
22n between the microphone and base of the audio amplifier, the
capacitor has a certain resistance at the frequency of the signal and
since this is audio, it has an effective resistance of about 4k. If you
put a 4k resistor in place of the 22n, you can see any signal produced
by the microphone is only a few kilo ohms away from the base of the
audio transistor. The audio transistor has an input impedance of about
4k and thus the two resistances can be seen as joined together in series
with the input of the transistor at their middle. They form a voltage
divider in which 50% of the signal produced by the microphone is
delivered to the transistor.
This is a very simple way to see the situation, so that if the 22n is
replaced by a 1n, very little of the signal produced by the microphone
will be delivered to the transistor.
But if the 22n is replaced by a 1u, abut 95% of the signal will be
delivered.
That's a choice you have to make. Experiment with the two values and see
if the improvement is noticeable.
When a capacitor is used to stabilize a voltage in a building block,
such as the 1n on the base of the oscillator, it is acting just like the
22n across the supply and it appears as tiny battery with a voltage of
about 2v and a resistance of about 2 ohms. This type of battery will
deliver 1 amp, so you can see the 1n will keep the base very stable.
The 10p to 47p coupling the oscillator to the output stage, is
equivalent to a very low resistor so nearly all the energy of the
oscillator is being passed to the output stage.
This is only a very simple way to look at the operation of each
capacitor but it gives an idea of why each value has been chosen.
It's a pity the designer of circuit #10 did not read these notes before
trying to design a kit for the electronics market.
GOING FURTHER
The next stage to improve the output,
matches the impedance of the output stage to the impedance of the
antenna.
The impedance of the output stage is about 1k to 5k, and the impedance
of the antenna is about 50 ohms.
This creates an enormous matching problem but one effective way is with
an RF transformer.
An RF transformer is simply a transformer that operates at high
frequency. It can be air cored or ferrite cored. The type of ferrite
needed for 100MHz is F28. The following circuit uses a small ferrite
slug 2.6mm dia x 6mm long, F28 material.
A slug is the screwed rod that screws into a coil and is adjusted to
change the matching of the windings or the frequency of the coil or
transformer.
To create an output transformer for circuit 6 above, wind 14 turns onto
the slug and 4 turns over the 14 turns. The ferrite core will do two
things. Firstly it provides a high amount of energy to pass from the
primary winding to the antenna. And secondly it will prevent harmonics
passing to the antenna.
The only way to prove the effectiveness of the transformer is with a
field test and the range increased nearly 100%, over the tuned output
design in circuit 9.
Matching the output to the antenna via a transformer
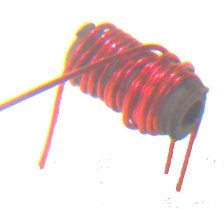
The output transformer
The photo above shows the 14 turns wound on the ferrite slug and 4 turns
wound over this winding. Remove the enamel from the 4 ends and solder
the leads as shown on the circuit diagram. It does not matter which way
the winding is wound and you can experiment with adding more turns to
the primary or removing turns from the secondary to get the highest
output.
A slight improvement to the design is to make an auto-transformer. The
circuit likes the fact that some of the current and voltage is already
flowing in the "secondary" of the auto-transformer and it simply adds to
this.
The other improvement is to make the output transistor self-biasing by
including an emitter resistor. This allows all the transistors in a batch
to take approximately the same current as the gain of each transistor
will determine the quiescent current, with high-gain devices taking more
current.
The addition of the 100R will reduce the spread in quiescent current.
The output of the stage will not fall since the impedance of the 1n at
100MHz is very small.
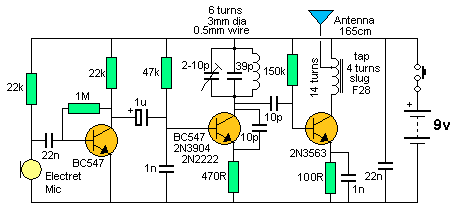
Using an Auto-transformer and self-biasing the output transistor
A transformer is the best way to couple the output of the transmitter to
the antenna but capacitors and inductors in an arrangement known as "pi,"
"T," or "L" coupling can be used. This is when you take advantage of the
fact that a capacitor can convert a high-voltage at low-current into a
low-voltage at high-current and an inductor has a higher impedance at
high frequency. It will reduce harmonics and the capacitor will provide
a higher current to produce higher electromagnetic radiation. This
is how a high impedance (resistance) is converted into a low impedance.
This type of coupling has been added to the following circuit.
This is actually highly effective but the components must be adjusted
accurately to have the greatest effect.
In addition, the frequency of the oscillator cannot be changed without
having to re-align the output.
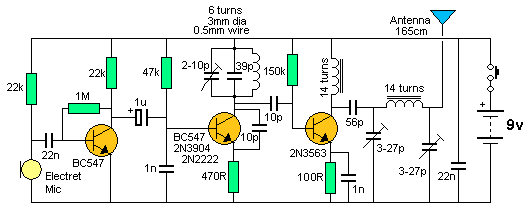
The following circuit uses three parallel tuned circuits to couple the
output of the transmitter to the antenna.
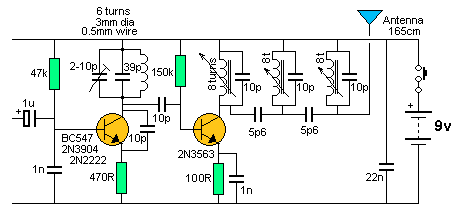
A triple-tuned output stage
Don't forget, you are trying to connect a 4k impedance of the circuit to
a 50 ohm load.
The first tuned circuit is in the collector of the output transistor. It is very similar to the
"inductor" circuit above except the capacitor across the coil will
improve the performance of the circuit enormously when the slug in the
coil is adjusted.
But this arrangement has a disadvantage. The
output will only perform to its best on a particular frequency (via the 10p
capacitor and 8t coil) when the slug in the coil is turned to produce a
maximum output.
The 2-10p air trimmer in the oscillator stage cannot be adjusted without
re-tuning the output stage and this limits the versatility of the
circuit.
However considerably more energy will be delivered to the antenna when the 3
tuned output stages are adjusted for maximum output. The signal from the
first is tapped off with a 5p6 capacitor to the second "TUNED
SECTION." This is the coil and capacitor in parallel.
The second "tuned section" is then adjusted to give maximum output. The
energy is then "tapped off" to a third tuned stage to which the antenna
is connected.
If you are prepared to have a fixed-frequency circuit, this arrangement
is excellent.
It does three things:
1. It separates the output stage from the antenna, You can touch
the antenna without affecting the frequency.
2. It converts more of the energy at the output stage, than previous
circuits, and
3. It matches the 4k of the output stage to the 50 ohm impedance of the
antenna.
Of course there is a lot of very complex mathematics that can be applied
to working out the
value if the components needed to interface between the output stage of a circuit and the antenna. But nowhere will you find the
"reason" why the components convert the signal from one impedance to
another.
For the first time we have told you: "it's the squeezing action
of the capacitor, converting a high-voltage, low-current into a
low-voltage high-current."
This produces the effect of converting a high impedance into a low
impedance.
An inductor can convert a low-voltage high-current into a high-voltage,
low-current by the collapsing magnetic field producing a very high
voltage and this is how we convert a low impedance into a high
impedance. You can read more about this in our project:
Solar Garden Light.
I am not going to argue with the pure mathematicians about this. I want
you to "see" how a circuit works so you can design anything and fix
it without having to get out a pencil and paper.
"All that matters in this world is to get something done, get paid, and
get on with the next thing." Leave the arguments 'till later.
THE COIL
Whenever you see a coil in a circuit, magic things can happen.
This is due to the phenomena of the coil producing a higher voltage (in
the opposite direction) when the applied voltage suddenly stops.
And when you see two windings (or one winding with an auto-transformer),
you do not know what output you will get without knowing all the details
of the transformer and actually seeing it in action.
The most effective oscillator coil has the dimensions of being at least
twice as long as its diameter.
In the following photo, the small coil is the TANK COIL and the larger
coil (called an inductor) is on the output.
The diameter of the small coil is too large. It has been wound on a 5mm
drill. It should be wound on a 3.5mm drill and the number of turns
increased from 4 to 6. (The turns are counted at the top of the coil.)
This will improve the "Q" of the coil. The inductor can also be reduced
in diameter.
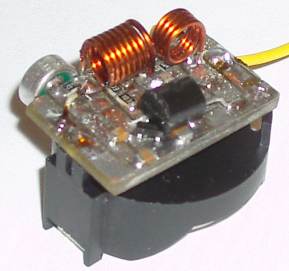
The diameter of the 4-turn TANK
COIL is too large
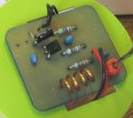
The turns are spaced too wide
The second image shows a 4-turn coil with
the turns spaced too wide. The maximum spacing is the thickness of the
wire.
When the turns are spaced very wide, the "Q" of the coil is reduced and
the performance of the circuit reduces.
When the length to diameter is 2:1, it allows the maximum amount of
magnetic flux surrounding the coil to collapse back and cut the turns of
the coil.
When designing a coil, here are the four most important features:
1. The most important feature is getting the length-to-diameter to be at
least 2:1
2. The next improvement is choosing the core material. Air has a value
of 1 at all frequencies. Ferrite (F29 material) will have a value
of about 3 at 100MHz. This means the number of turns can be reduced to
achieve the same inductance, and the coil will be less prone to external
influences such as metal objects, but we have not found the output to be
any better than an air coil (in any of the FM transmitters we have
designed).
3. The next critical factor is the spacing of the turns. The best is
close-spaced but they can have a small gap (the width of the wire) and
the coil will still be effective.
4. The gauge of the wire is not particularly critical however wire
thinner than 0.25mm will vibrate and produce microphonic sounds. 0.5mm
wire is the maximum you need. Thicker than 0.5mm is not needed.
0.1uH COIL
On some circuits you will find a value of 0.1uH (100nH) for the coil. If
you buy a ready-made inductor of this value, the circuit may work or it
may not. The reason is the coil in the TANK circuit must have a high
value of "Q" to get the greatest range. To achieve a high Q, the coil
should be hand-wound as shown in the notes for the circuits. A
ready-made inductor will be many turns of very fine wire and will not be
in the ratio mentioned above. The wire will also be too fine. In. In
addition, a hand-made coil can be adjusted by stretching the turns.
THE ANTENNA
The secret to getting a good
range with low power is providing a good transmitting antenna.
In addition, a good ground-plane is also needed. The batteries are
normally used to provide a ground-plane and that's why the leads to the
battery must be kept as short as possible. 5cm of battery lead can
reduce the output to half. Connecting the battery directly to the board
can double the output. Adding a thick ground-plane to the PC board can
also help considerably.
But, of course, it is the antenna that does most of the work.
Since we are transmitting at about 100MHz, the wavelength of the signal
is 3.3 metres and
a full wave antenna would be 3.3 metres long.
It is often quite inconvenient to use a long antenna, so here are
some alternatives.
Obviously you can use a shorter antenna, but as you reduce the length,
the range reduces.
Surprisingly, it does not decrease greatly when the antenna is reduced
to "half-wave" and this is what we have supplied with all Taking
Electronics FM transmitters.
Many of the kits on the web are supplied with an antenna 30cm long to
reduce the range and make the transmitter somewhat legal. But this is
not what building and testing is all about.
The hobbyist wants to know how far he can reach with the transmitter he
has made.
Placing the antenna on top of a cupboard is a good choice. It provides
height and you can stretch the antenna to its full 1.65m length.
The receiving antenna on a radio must also be horizontal and somewhere
at the front or back of the transmitting antenna.
If you require omni directional radiation, (radiation 360°)
the antenna must be upright (vertical). The receiving antenna must also
be vertical and can be anywhere in the 360°
radius.
We are not going into the complexities of different radiating patterns
of antennas but here is one suggestion for an improved antenna. It is
called a DIPOLE. You can get a closed dipole, folded dipole and
others but ours is a simple design.
THE DIPOLE
If you want to transmit in a particular direction, you can fit a dipole
antenna..
This is simply connecting one wire to the antenna output and another
wire to the 0v rail and stretching each out horizontally in the opposite
directions.
These are called RADIATORS and if you want to have them higher than the
transmitter, the two wires run parallel to each other and very close.
These are called FEEDERS.
Here is a diagram of a DIPOLE:
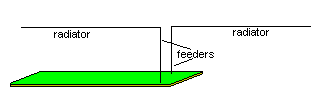
A Dipole antenna
This will produce an antenna over 3 metres
long and the transmission will be greatest at the front and back.
You can experiment by shortening both wires and do a field test to
determine the range.
Adding a wire to the 0v rail increases the output as it gives the
circuit a good ground plane and allows more signal to be pushed out the
"antenna point" (on the circuit).
The feeder wires do not radiate a signal because each line is close to
the other and the signals cancel. That's why the feeder wires should not
be too close - they lose energy and if they are close together, they
lose more energy and they should be short as possible.
MORE POWER
Everyone wants to transmit as far
as possible and build the most powerful transmitter in the world.
But you have to be careful.
An FM transmitter can reach an enormous distance with just a few
milliwatts and when you start building one with a consumption
of 1watt or more, you are getting into serious territory.
We produce a 30mW bug called the AMOEBA and it was tested by a hobbyist
on top of a mountain and it transmitted 40km (26 miles) to his home. We
regularly get 400m in a built-up area, so you can see, hundreds of
people in the 400m radius could be detecting the channel.
You can imagine how far a 1watt transmitter will travel and how many
people could be involved.
But it is not just the wattage. When you design a powerful transmitter, you
must be very careful to eliminate unwanted harmonics. These are
frequencies that are generated by the circuit (and get radiated with the
signal) to produce all sorts of havoc. They are at a lower power-level than the
main frequency but they are very difficult to detect.
A powerful transmitter can produce a lot of these
and nearby neighbours can get very upset if your device interferes with
their baby monitor or radio reception or home intercoms etc.
Since I live near a training airport, I have to be very careful not to
produce anything that might upset the communications between the tower
and training aircraft.
Airport channels and emergency channels are very close to the
frequencies we are using and when a transmitter gets into the 1watt category, you don't know who you may be upsetting.
The circuits we have presented to date are less than 50mW output and
very little real interference can be produced.
The next 3 circuits are for experimental purposes and have an output of about 200mW to 400mW.
A circuit diagram does not show the screening necessary to keep the
harmonics to a very low level. You need to see a photo of the unit
and how the components have been laid out.
The construction and placement of the filtering components on the output
is also important.
That's why these circuits are more complex than just putting the
components together and connecting a supply.
200mW FM
TRANSMITTER
The power output of many transmitter circuits are very low because no
power amplifier stages are incorporated. The transmitter circuit
described here has an extra RF power amplifier stage, after the
oscillator stage, to raise the power output to 200-250 milliwatts. With
a good matching 50-ohm ground plane antenna or multi-element Yagi
antenna, this transmitter can provide reasonably good signal strength up
to a distance of about 2 kilometres.
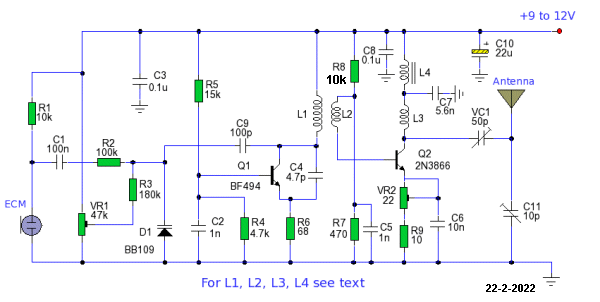
Circuit Notes
The circuit is built around transistor T1 BF494. It
is a low-power variable-frequency VHF oscillator. A varicap diode
is included to change the frequency of the transmitter and to
provide frequency modulation of the audio signals. The output of the
oscillator is about 50 milliwatts. Transistor T2 2N3866
forms a VHF
"class A" power amplifier. It boosts the oscillator signal
power four to five times. Thus, 200-250 milliwatts of power is provided
at the collector of transistor T2.
For best results, construct the circuit on a PC board with all
components closely connected and put the transmitter in an
aluminum case.
Coil winding details::
L1 - 4 turns of 20 SWG wire close wound on 8mm diameter plastic
former.
L2 - 2 turns of 24 SWG wire near top end of L1.
(Note: air core for the above coils)
L3 - 7 turns of 24 SWG wire close wound with 4mm diameter air core.
L4 - 7 turns of 24 SWG wire-wound on a ferrite bead (as choke)
-
Potentiometer VR1 is used to vary the fundamental
frequency whereas potentiometer VR2 is used as power control.
-
For hum-free operation, connect the transmitter
on a 12V rechargeable battery pack of 10 x 1.2-volt Ni-Cd cells.
-
Transistor T2 must be mounted on a heat sink.
-
Do not switch on the transmitter without a
matching antenna.
-
Adjust both trimmers (VC1 and VC2) for maximum
transmission power.
-
Adjust potentiometer VR1 to set the
frequency to about 100MHz.
POWERING A BUG
It is always the best to power a bug
from a battery. It keeps the whole project simple.
However if you want to power a bug for a long period of time, here is a
circuit
that will reduce the hum from a normal plug pack by a factor of about
2,000.
This means a 20mV ripple will be 1uV and will not
be noticed. Any background hum is annoying and very difficult to remove
with electrolytics. This circuit is the answer. The 1k and 100u form a
filter that makes the 100u ten times more effective than if
placed directly on the supply-line. The transistor reduces the ripple by
a factor equal to the gain of the transistor - about 200. The result is
a reduction by a factor of 2,000. The circuit is suitable for up to
100mA. A power transistor can be used, but the 1k will have to be
reduced to 220R for 500mA output. The output of the circuit is about
0.6v less than the output of the plug pack.
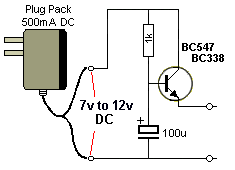
An ELECTRONIC FILTER
P1 P2
P3
228/3/2013
|